Strain Improvement (LANL)
In DISCOVR, our goal is to use non-genetic modification strategies to improve the phenotypes of a subset of our Tier III strains. We employ approaches such as traditional adaptive laboratory evolution, random mutagenesis, and flow cytometry. Flow cytometry has been a core capability at Los Alamos National Laboratory for over 30 years. Although possibly best known for its clinical applications, flow cytometry is in essence a tool for the high throughput (thousands per second) screening of small particles. At LANL, we focus on developing a range of new applications in flow cytometry across a variety of cell types. In biofuels and bioproducts, our aim is to identify and isolate the best performing cells in a culture population, where performance might be lipid or other product accumulation, cell growth, cell health, or other related phenotype.
To date, our main focus in using adaptive evolution has been for the improvement of salinity tolerance in strains of interest. Several of the best performing strains isolate from the Tier I and II screens in DISCOVR perform best in freshwater media. However, our goal is to grow cultures outdoors in brackish to marine salinities, to avoid competition with freshwater resources. Building off of previous work in Chlorella, in DISCOVR we used serial passaging to improve the growth rate of Scenedesmus obliquus UTEX393 in a 15 ppt salinity medium (Figure 1). We have also determined that the adapted culture has an ~30% increase in percent fatty acid methyl esters (FAMEs) per ash free dry weight of biomass, over the freshwater culture under nitrogen deplete conditions. We have also treated this strain with ultraviolet light to generate genetic mutations that may confer additional improved productivity phenotypes.
For flow cytometry-based research, we have a suite of five core cytometers, with access to three others. These range from benchtop ‘workhorse’ cytometers such as the BD Accuri C6, to an Amnis Imagestream X imaging flow cytometer, to several fluorescence-activated cell sorters (FACS). Each of these instruments has a unique set of functions for tracking or isolating different algae phenotypes.
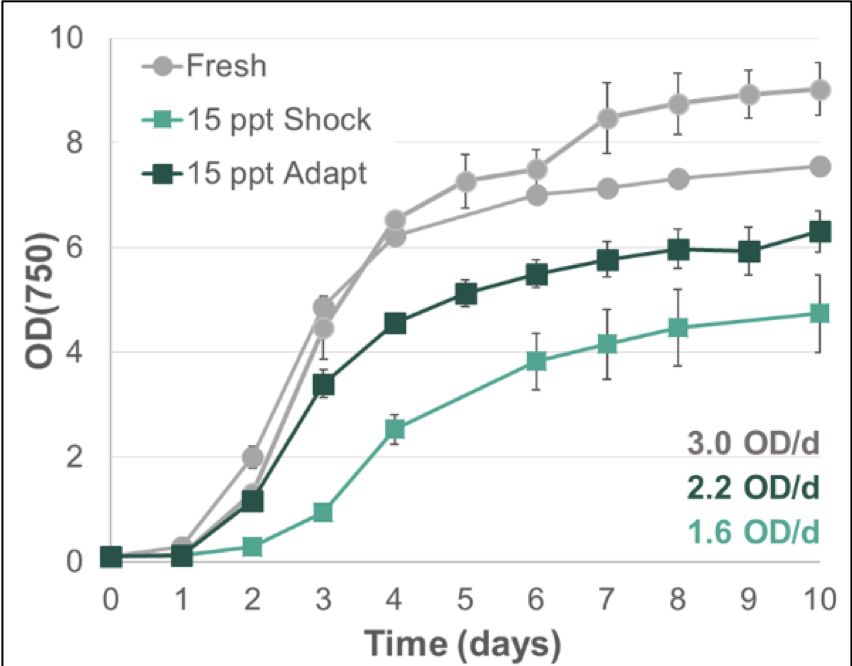
Figure 1. Adaptation of Scenedesmus obliquus UTEX393 to increased salinity
To date, our main focus in using adaptive evolution has been for the improvement of salinity tolerance in strains of interest. Several of the best performing strains isolate from the Tier I and II screens in DISCOVR perform best in freshwater media. However, our goal is to grow cultures outdoors in brackish to marine salinities, to avoid competition with freshwater resources. Building off of previous work in Chlorella, in DISCOVR we used serial passaging to improve the growth rate of Scenedesmus obliquus UTEX393 in a 15 ppt salinity medium (Figure 1). We have also determined that the adapted culture has an ~30% increase in percent fatty acid methyl esters (FAMEs) per ash free dry weight of biomass, over the freshwater culture under nitrogen deplete conditions. We have also treated this strain with ultraviolet light to generate genetic mutations that may confer additional improved productivity phenotypes.
For flow cytometry-based research, we have a suite of five core cytometers, with access to three others. These range from benchtop ‘workhorse’ cytometers such as the BD Accuri C6, to an Amnis Imagestream X imaging flow cytometer, to several fluorescence-activated cell sorters (FACS). Each of these instruments has a unique set of functions for tracking or isolating different algae phenotypes.
In the DISCOVR project, we focus primarily on two cell sorting approaches, both of which rely on a fluorescence read-out from the cells that is correlated to performance (Figure 2). First, we use our adaptation of cell sorting for an improved lipid phenotype using the neutral lipid stain, BODIPY™ 505/515. Cells from the relevant algae culture are stained with BODIPY, and the most fluorescent cells are isolated using FACS. After multiple rounds of sorting, a stable higher lipid-accumulation subpopulation can be acquired and maintained. Second, we utilize a gel microdroplet based approach to isolate the fastest dividing cells in the culture. This latter method relies on the natural autofluorescence of the cells to track and sort cells.
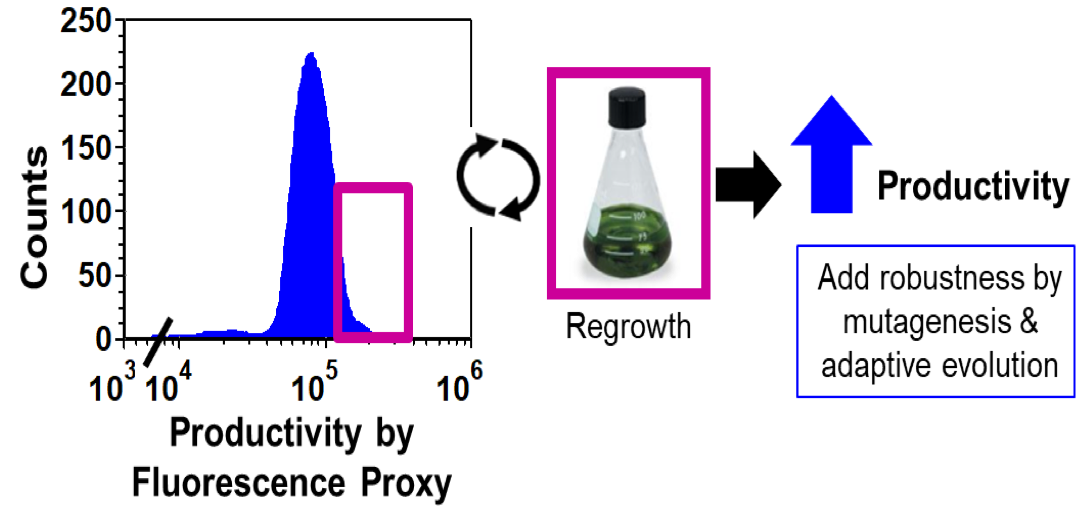
Figure 2. General schematic for strain improvement using flow cytometry.
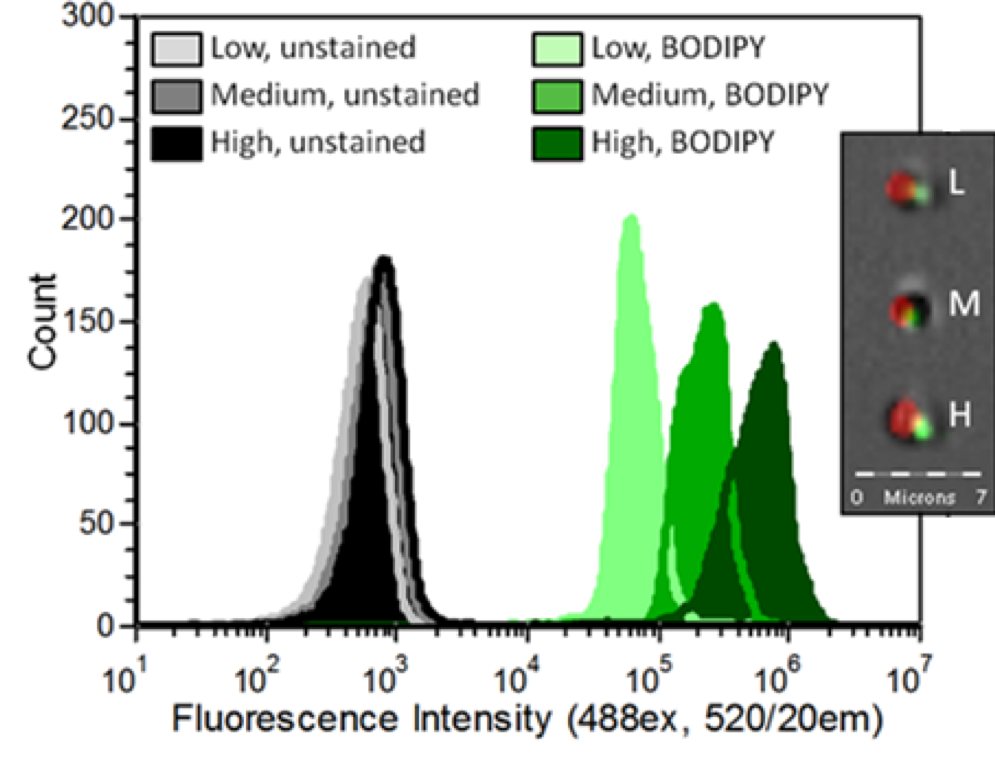
Figure 3. Observation of changes in neutral lipid accumulation after nitrogen starvation of Picochlorum soloecismus.
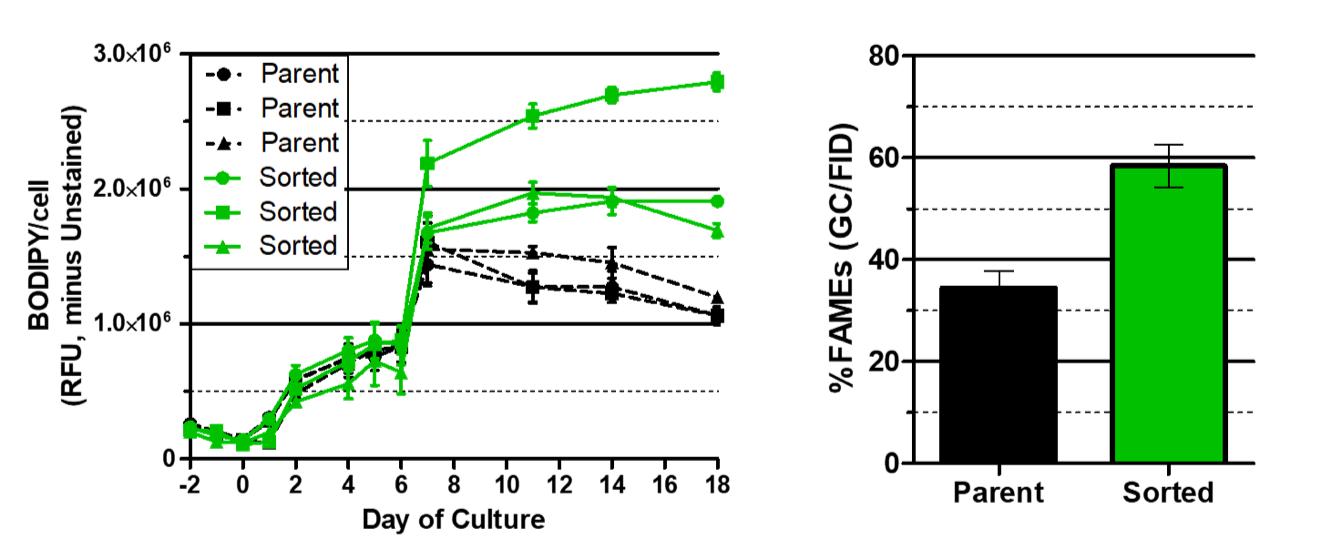
Figure 4. Comparison of lipid accumulation over time in a parent population and cell sorted population by BODIPY, and end point measurement of percent FAME per dry weight for the same cultures
Culture analysis using flow cytometry can provide a rapid (same-day) evaluation of culture health and performance. In a single measurement, we can track cell size and relative granularity, chlorophyll autofluorescence, and lipid content (Figure 3). Cell number is also a standard measurement. These measurements give an in-depth, single-cell understanding of strain performance in a given system, which complements more bulk measurements such as optical density, ash free dry weight, and biochemical composition.
Cell sorting for strain improvement typically results in a subpopulation with an increased BODIPY staining phenotype. Because an increase in fluorescence could mean the isolation of cells that simply take up the dye more efficiently, or the isolation of larger cells with larger or more lipid bodies per cell, we also measure the actual percent FAMEs in cultures of the isolated subpopulations by gas chromatography with flame ionization detection (GC/FID, Figure 4).
Using flow cytometry as a culture screening or strain improvement method has the benefits of relatively rapid characterization of culture performance and permitting the isolation of improved phenotypes without genetic modification. This approach can be coupled to random mutagenesis approaches, such as chemical or UV mutagenesis, as well as coupled to traditional adaptive evolution techniques.
Huesemann M, Dale T, Chavis A, Crowe B, Twary SN, Barry A, Valentine D, Yoshida R, Wigmosta M (2017). Simulation of outdoor pond cultures using indoor LED-lighted and temperature controlled raceway ponds and Phenometrics™ photobioreactors. Algal Research, 21, 178-190.
Olivares J, Unkefer CJ, Sayre RT, Magnuson JK, Anderson DB, Baxter I, Blaby IK, Brown JK, Carleton M, Cattolico R, Dale T, et al. (2017). Review of the algal biology program within the national alliance for advanced biofuels and bioproducts. Algal Research. http://dx.doi.org/10.1016/j.algal.2016.06.002.
Bagwell CE, Abernathy A, Barnwell R, Milliken CE, Noble PA, Dale T, Beauchesne KR, Moeller PD (2016). Discovery of bioactive metabolites in biofuel microalgae that offer protection against predatory bacteria. Frontiers in Microbiology, 7, article 516, 1-12.
Strain improvement, non-GMO, adaptive evolution, mutagenesis